The Biological Substrate of the Motoric Cognitive Risk Syndrome: A Pilot Study Using Amyloid-/Tau-PET and MR Imaging
Abstract
We conducted a cross-sectional pilot study to explore the biological substrate of the Motoric Cognitive Risk (MCR) syndrome in a Memory Clinic cohort, using a multimodal imaging approach. Twenty participants were recruited and classified as MCR+/−. Amyloid- and tau-PET uptakes, temporal atrophy, white matter hyperintensities, lateral ventricular volume (LVV), and diffusion tensor parameters were compared between groups. No significant differences were found in imaging features related to Alzheimer’s disease or gross vascular damage. MCR+ patients had increased LVV and altered diffusion parameters in the superior corona radiata. Ventricular enlargement and microstructural damage of the surrounding white matter tracts could contribute to MCR pathophysiology.
INTRODUCTION
Motoric Cognitive Risk (MCR) syndrome is a pre-dementia condition based on the presence of cognitive complaints and slow gait [1]. It has a worldwide prevalence of around 10%,in older adults and a predictive validity for incident dementia and adverse clinical outcomes, such as falls or mortality [2]. Pathophysiological mechanisms underlying MCR are probably heterogeneous and have not been fully established yet. Indeed, MCR has been variably related to vascular risk factors [3, 4], increased levels of systemic inflammatory biomarkers [5], cortical atrophy [6, 7], cerebrovascular lesion load [8], and to an increased risk of developing vascular dementia or Alzheimer’s disease (AD) [1, 9, 10]. A recent study revealed an association between APOE ɛ4 and the risk of developing dementia in patients with MCR [11]. Yet, AD biomarkers (i.e., amyloid and tau deposition, and neurodegeneration), as well as indicators of underlying pathology other than atrophy or vascular damage, have not been assessed in MCR.
In this exploratory study, we used a multimodal imaging approach to investigate the pathophysiology underlying MCR in a cohort of patients referring to the Memory Clinic. Specifically, we evaluated whether amyloid and tau deposits, white matter changes, and features indicative of neurodegeneration or microstructural damage in gait-related tracts were different between patients with (MCR+) and without (MCR–) MCR.
MATERIALS AND METHODS
Participants
Twenty older adults (mean age 73.2±6.4 years, 7 females) referred to the Memory Center of the Geneva University Hospitals for cognitive complaints were prospectively recruited from July 2020 to January 2021. Exclusion criteria were the presence of 1) major neurocognitive disorder/dementia (as defined by the diagnostic and statistical manual of mental disorders (DSM-V), 2) neurological and psychiatric disorders other than cognitive impairment, and 3) gait impairment caused by orthopedic, rheumatologic, or other severe medical condition.
Patients underwent a clinical and gait assessment, an amyloid and tau PET, and MR brain imaging, including a T1-weighted, a fluid-attenuated inversion recovery (FLAIR) and an echo planar diffusion tensor imaging (DTI) sequence.
This study was approved by the local ethics committee and all participants provided written informed consent prior to participation.
Clinical assessment
Demographic and clinical data were collected, including education level (as a categorical variable, defined as I: < 9 years, II: 9–12 years, and III: > 12 years), neuropsychological tests, vascular risk factors, and Clinical Dementia Rating (CDR) scale score. APOE genotype (determined using real-time TaqMan assay (Applied Biosystems)) was available for 11 out of 20 patients.
Gait assessment and MCR status
Gait speed during normal walking was measured using a 12-camera optoelectronic system (Oqus7+, Qualisys, Sweden). MCR status was defined by a gait speed value ≤ [mean value − 1 standard deviation] in a cohort of patients with cognitive complaints, with respect to the appropriate age- and gender-matched class values [1]. Reference values were derived from a local cohort of healthy older adults, assessed using the same gait protocol (reported in the Supplementary Material).
PET
Acquisition and preprocessing of PET data are reported in the Supplementary Material. The standardized uptake value ratio (SUVr) for amyloid and tau images were obtained as in [12] and [13], using the whole cerebellum and the cerebellum crus as reference regions, respectively. Given that Amyloid-PET was acquired using different ligands, in line with established research practice in the field, we converted SUVRs into Centiloid, a measure developed to scale the outcome of each particular ligand uptake to a common 0 to 100 scale, as recommended in [14]. Amyloid positivity was determined (i) visually from a nuclear medicine specialist and (ii) using previously established Centiloid unit cut-off of 26. Visual and automated assessment was concordant in all cases. Tau PET positivity was determined using the previously established SUVr cut-off of 1.25 [13].
MRI
All patients underwent MRI on a Siemens MAGNETOM Skyra 3T. Sequence parameters and further details on images processing are detailed in the Supplementary Material.
White matter hyperintensities (WMH)
Lesions were segmented from FLAIR sequences and the total lesion volume was extracted.
Volumetric measures
T1-weighted sequences were segmented using Freesurfer 6.0.0. Lateral ventricular volume (LVV) and the estimated total intracranial volume were derived. Values from the entorhinal, fusiform, inferior temporal, and middle temporal regions were averaged to obtain a temporal region of interest (ROI) thickness [17]. Thickness values from other cortical regions previously related to MCR, specifically prefrontal, insular, temporal, and parietal regions [6, 7], were also extracted and are available in the Supplementary Material.
DTI processing
Data were available for 19 out of 20 subjects. Given the exploratory nature of the study, we chose to focus on white matter tracts related to gait, based on data available in literature [15, 16], and specifically on the cortico-spinal tract (CST), the corpus callosum (CC), and superior corona radiata (sCR). However, DTI parameters of other relevant tracts are reported in the Supplementary Material. Mean fractional anisotropy (FA), mean diffusivity (MD), axial diffusivity (AxD), and radial diffusivity (RD) values were extracted from each ROI for each subject.
The following imaging features were considered for analyses (Fig. 1): 1) amyloid and tau status (positive/negative), amyloid PET Centiloid, tau PET SUVr, and the medial-basal-lateral temporal lobe atrophy [17] as AD biomarkers; 2) WMH volume to assess vascular damage; 3) LVV, normalized for total intracranial volume, and 4) MD and FA of the CC, CST, and sCR, as indices, respectively, of unspecific neurodegeneration and white matter microstructural damage, previously described as contributors to impaired gait [15, 16, 18–20].
Fig. 1
Representative images of a patient for each PET and MR imaging modality used and violin plots of the respective imaging features in MCR+ and MCR–. SUVr, standardized uptake value ratio; T1w, T1-weighted sequence; LVV, lateral ventricles volume; FLAIR, fluid-attenuated inversion recovery; WMH, white matter hyperintensities; DTI, diffusion tensor imaging; FA, fractional anisotropy; MD, mean diffusivity; AxD, axial diffusivity; sCR, superior corona radiata; CC, corpus callosum; CST, cortico-spinal tract. *p < 0.05.
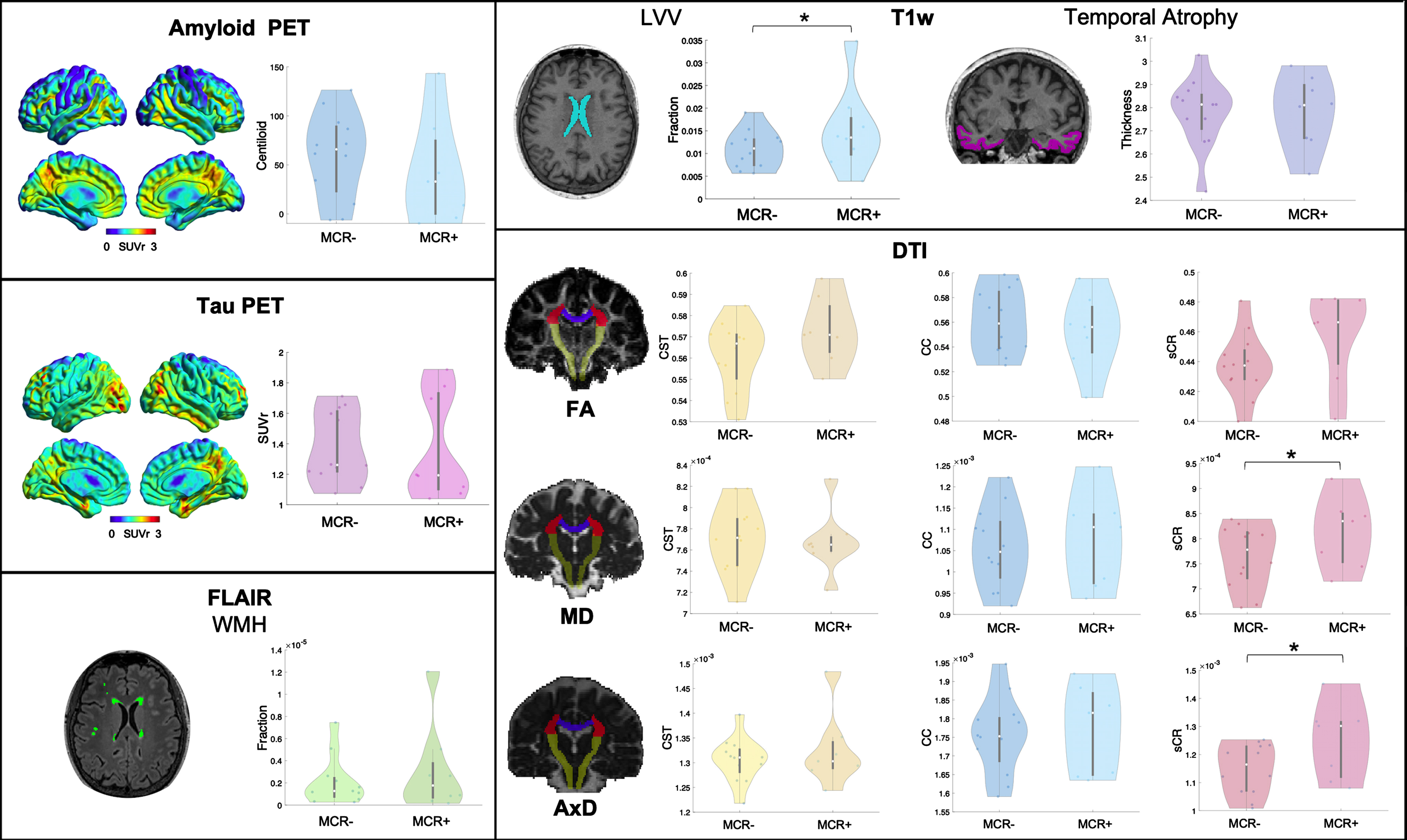
Statistical analysis
Wilcoxon rank sum test and chi-squared were used to compare demographic and clinical characteristics and amyloid or tau status between the MCR+/–groups. A Quade non-parametric ANCOVA analysis, using age as covariate, was performed to compare amyloid Centiloid, tau SUVr, and MRI parameters between the two groups. Effect sizes were computed and reported as Phi, r, or f, where a Phi or r (f) value from 0.1 to 0.30 (0.25) indicates a small effect, from 0.30 (0.25) to 0.50 (0.40) a medium effect, and > 0.50 (0.40) a large effect size.
Data availability
Data supporting the findings of this study will be shared upon request.
RESULTS
Demographic and clinical data of the whole cohort and separately for MCR+ and MCR–groups are reported in Table 1. All patients had a CDR scale score of 0.5. The 8 MCR+patients (40%,) did not differ in terms of demographic or cognitive performance from the 12 MCR–patients. Results for imaging metrics are reported in Table 2 and Fig. 1. Amyloid or tau uptake, temporal ROI thickness, WMH volume and CST or CC microstructural damage did not differ between the two groups. The LVV and the MD, AxD, and RD of sCR were significantly higher in MCR+ compared to MCR–(F(1,18) = 5.92, p = 0.026, F(1,17) = 9.40, p = 0.007, F(1,17) = 9.68, p = 0.006 and F(1,17) = 4.60, p = 0.047, respectively).
Table 1
Demographic and clinical data of the whole cohort and MCR+ and MCR–patients
All patients (n = 20) | MCR+ (n = 8) | MCR– (n = 12) | p | Effect size | |
Age (y) | 74.0 (7.0) | 73.5 (3.0) | 74.5 (10.5) | 0.535 | 0.14 |
Gender (n of females/males) | 7/13 | 2/6 | 5/7 | 0.444† | 0.17† |
Time from symptoms onset (months) | 45.2 (63.0) | 46.5 (62.9) | 39.9 (75.4) | 0.758 | 0.07 |
Education level (n of patients with level I/II/III) | 2/5/13 | 1/2/5 | 1/3/8 | 0.953† | 0.07† |
MMSE | 27.0 (3.0) | 27.0 (2.5) | 27.0 (2.8) | 0.379 | 0.20 |
Ten-point clock test | 9.0 (3.5) | 9.0 (5.0) | 8.5 (3.0) | 0.843 | 0.05 |
FCSRT-TR score | 40.0 (6.3) | 38.0 (3.0) | 43.0 (5.5) | 0.174 | 0.33 |
Digit span score | 6.0 (2.0) | 6.0 (3.0) | 6.0 (2.0) | 0.605 | 0.12 |
TMT-B/TMT-A ratio score | 2.3 (0.7) | 2.5 (0.5) | 2.3 (1.1) | 0.856 | 0.05 |
Vascular risk factors (n, %,) | |||||
Hypertension | 9 (45%,) | 4 (50%,) | 5 (41.7%,) | 0.714† | 0.04† |
Diabetes | 2 (10%,) | 1 (12.5%,) | 1 (8.3%,) | 0.761† | 0.04† |
Hypercholesterolemia | 8 (40%,) | 4 (50%,) | 4 (33.3%,) | 0.456† | 0.08† |
Smoking | 2 (10%,) | 1 (12.5%,) | 1 (8.3%,) | 0.761† | 0.04† |
Cardiovascular disease | 4 (20%,) | 2 (25%,) | 2 (16.7%,) | 0.648† | 0.05† |
APOE genotype available (n, %,) | 11 (55%,) | 4 (50%,) | 7 (58.3%,) | ||
ɛ4 carriers | 3 (27.3%,) | 0 | 3 (42.9%,) | 0.125† | 0.22† |
WS (m/s) | 1.10 (0.22) | 0.98 (0.08) | 1.18 (0.20) | 0.003 | 0.67 |
Time from symptoms onset is referred to the time lag between onset of cognitive complaints as reported by the patients and the date of assessment. Education level is determined as follows: I: < 9 years, II: 9–12 years, and III: > 12 years; MCR, motoric cognitive risk; MMSE, Mini-Mental State Examination, higher scores indicate a better performance; FCSRT-TR, Free and Cued Selective Reminding Test-Total Recall; TMT-A, Trail Making Test part A; TMT-B, Trail Making Test part B; WS, walking speed. Values are expressed as median (interquartile range) unless otherwise specified. The reported p-values are derived from chi-squared test for categorical variables (†) or from non-parametric Wilcoxon rank sum test. Effect sizes are reported as Phi (†) or r, where a Phi (r) value from 0.1 to 0.30 indicates a small effect, from 0.30 to 0.50 a medium effect, and > 0.50 a large effect size.
Table 2
Imaging parameters in the whole sample and the MCR+ and MCR- groups
All patients (n = 20) | MCR+ (n = 8) | MCR– (n = 12) | p | Effect size | |
Amyloid positive (n, %,) | 13, 65%, | 5, 62.5%, | 9, 75%, | 0.550† | –0.13† |
Tau positive (n, %,) | 9, 45%, | 3, 37.5%, | 6, 50%, | 0.582† | –0.12† |
Amyloid PET Centiloid | 59.4 (77.6) | 33.0 (76.3) | 65.9 (67.8) | 0.522 | 0.15 |
Tau PET SUVr | 1.25 (0.49) | 1.19 (0.64) | 1.26 (0.41) | 0.680 | 0.10 |
Total ICV (x106) | 1.51 (0.10) | 1.57 (0.10) | 1.49 (0.17) | 0.070 | 0.89 |
WMH volume (mL) | 1.79 (3.32) | 2.43 (5.75) | 1.79 (2.99) | ||
WMH volume fraction (x10–6) ‡ | 1.27 (2.07) | 1.77 (3.26) | 1.27 (1.85) | 0.749 | 0.08 |
LVV mL | 19.0 (11.0) | 21.7 (12.4) | 17.1 (11.7) | ||
LVV fraction (x10) ‡ | 0.12 (0.07) | 0.14 (0.08) | 0.11 (0.06) | 0.026 | 0.57 |
Temporal ROI cortical thickness | 2.81 (0.21) | 2.81 (0.24) | 2.81 (0.15) | 0.961 | 0.01 |
CC FA | 0.56 (0.04) | 0.56 (0.04) | 0.56 (0.05) | 0.274 | 0.25 |
MD (×10–3 mm2 s–1) | 1.06 (0.16) | 1.11 (0.17) | 1.05 (0.14) | 0.253 | 0.27 |
AxD (×10–3 mm2 s–1) | 1.76 (0.18) | 1.82 (0.22) | 1.75 (0.12) | 0.239 | 0.29 |
RD (×10–3 mm2 s–1) | 0.71 (0.15) | 0.75 (0.15) | 0.69 (0.14) | 0.453 | 0.19 |
sCR FA | 0.44 (0.04) | 0.47 (0.04) | 0.44 (0.02) | 0.059 | 0.49 |
MD (×10–3 mm2 s–1) | 0.80 (0.10) | 0.84 (0.10) | 0.78 (0.10) | 0.007 | 0.72 |
AxD (×10–3 mm2 s–1) | 1.21 (0.17) | 1.30 (0.21) | 1.16 (0.16) | 0.006 | 0.73 |
RD (×10–3 mm2 s–1) | 0.58 (0.06) | 0.60 (0.04) | 0.57 (0.07) | 0.047 | 0.50 |
CST FA | 0.57 (0.02) | 0.57 (0.02) | 0.57 (0.02) | 0.172 | 0.35 |
MD (×10–3 mm2 s–1) | 0.77 (0.04) | 0.77 (0.01) | 0.77 (0.05) | 0.899 | 0.05 |
AxD (×10–3 mm2 s–1) | 1.31 (0.04) | 1.30 (0.06) | 1.31 (0.05) | 0.429 | 0.18 |
RD (×10–3 mm2 s–1) | 0.50 (0.04) | 0.50 (0.01) | 0.50 (0.05) | 0.868 | 0.05 |
MCR, motoric cognitive risk; SUVr, standardized uptake value ratio; ICV, intracranial volume; WMH, white matter hyperintensities; LVV, lateral ventricles volume; ROI, region of interest including the entorhinal, fusiform, inferior temporal and middle temporal regions; CC, corpus callosum; sCR, superior corona radiata; CST, cortico-spinal tract; FA, fractional anisotropy; MD, mean diffusivity; AxD, axial diffusivity; RD, radial diffusivity. Higher Amyloid Centiloid and PET SUVr values indicate increase in amyloid and tau deposits, respectively. Decreased FA and increased MD are markers of white matter injury. Changes in RD and AxD can reflect altered myelination, axonal damage or stretching/compression. ‡ fraction is expressed as: LVV/estimated total intracranial volume. Values are expressed as median (interquartile range), unless otherwise specified. The p-values reported are derived from chi-squared test for categorical variables (†) or Quade nonparametric ANCOVA analysis with age as covariate. Effect sizes are reported as Phi (†) or f, where a Phi (f) value from 0.1 to 0.30 (0.25) indicates a small effect, from 0.30 (0.25) to 0.50 (0.40) a medium effect, and > 0.50 (0.40) a large effect size.
Additional cortical thickness and DTI parameters values are available in the Supplementary Material. No other regional thickness resulted significantly different between the two groups, while the AxD of the anterior limb of internal capsule was significantly higher in the MCR+ group.
DISCUSSION
In this pilot study we investigated the pathophysiological substrate of MCR in a Memory Clinic cohort, using a multimodal imaging approach. We assessed whether biomarkers of AD, vascular disease, gait-related white matter tracts damage, or other indicators of unspecific neurodegeneration were associated with MCR syndrome. Our results disclosed 1) no significant differences in imaging biomarkers of AD, i.e., amyloid or tau deposition and temporal atrophy, or gross vascular damage, i.e., WMH, between MCR- and MCR+ groups, and 2) larger LVV and increased MD, AxD, and RD values at the level of the sCR in MCR+ patients.
So far, studies have discordantly associated MCR with an increased risk of vascular dementia, AD, or both. In this exploratory study, we concurrently investigated the relationship between MCR and imaging biomarkers for both AD and vascular disease, finding no significant differences in terms of amyloid and tau burden or gross vascular pathology between MCR+ and MCR- patients. Although this result could be ascribable to the small sample size, it is in line with previous studies, suggesting a not exclusive association between MCR and a unique neurodegenerative disorder.
Previous studies found a correlation between gait parameters and amyloid deposition in subcortical and cortical areas, while a recent study did not find an increased amyloid PET SUVr in MCR+ patients [21]. Here, for the first time, we also compare tau PET SUVr between MCR+ and MCR- patients, finding no differences. Tau deposits assessed with PET imaging have been shown to better correlate to clinical status and to predict cognitive decline in AD [22]. The absence of an increase in tau burden confirms that MCR is probably not due to a pure AD pathology.
Although MCR has been initially described as a syndrome predictive of vascular dementia [1] and WMH are widely reported in MCR cohorts, a clear association between WMH and MCR is lacking, with previous studies reporting an increased rate of frontal lacunar infarcts, but not of the global or regional WMH loads in patients with MCR [23]. This could suggest that a spatial pattern of damage, more than the underlying mechanism causing it, would explain MCR. Such spatial pattern would be better captured with measures of atrophy or white matter microstructural damage. Indeed, volumetric measures of grey matter regions involved in gait control have been related to MCR.
In this work, the larger LVV and sCR changes we observed in MCR+ patients reveal the potential contribution of ventricular enlargement, and the damage of adjacent white matter tracts involved in gait control, to MCR pathogenesis. The significant difference observed for the AxD of the anterior limb of internal capsule, another tract involved in gait control [24, 25] and adjacent to lateral ventricles, goes in the same direction. Ventricular volume and altered white matter microstructure have been already associated with slow gait [15, 18, 20]. Moreover, the sCR includes projections from prefrontal and supplementary motor cortices and fronto-insular connections [26], which are regions found to be atrophic in MCR [6], thus further supporting the involvement of such brain areas in this pre-dementia syndrome. It is noteworthy the increase in MD, AxD, and RD, associated to a not significant change in FA (possibly explained by the concomitant increases of both AxD and RD), observed in our cohort. The same findings have been detected in periventricular fibers of patients with normal pressure hydrocephalus, a condition highly prevalent in older adults. Moreover, changes in DTI values correlate with ventricular volume in healthy subjects and patients with hydrocephalus [27]. Therefore, microstructural changes and enlarged ventricles may represent two aspects of the same pathological mechanism. Possible explanations include neuronal damage and loss, decrease in axon density and axonal stretching. In particular, while MD changes are more sensitive to vascular damage [28] but aspecific to the pathogenic mechanism, the increase in AxD, which probably drives the MD change, may be related to axonal stretching and compression, due to ventricular enlargement. However, a reduced axon density and irreversible neuronal damage are also putative mechanisms underlying such alterations in DTI parameters [29]. Further studies are needed to determine whether they are expression of unspecific neurodegeneration or altered cerebrospinal fluid dynamic as occurring in hydrocephalus.
This work comes with some limitations. The first is small sample size: although we conceived this as a pilot study, the cohort size limits its impact and the statistical power, and results need to be confirmed in larger samples. A second limitation of this study is the absence of a group of healthy controls, since MCR- patients reported cognitive complaints and presented with a CDR score of 0.5. This approach has been previously used to investigate imaging features in MCR [7] and it reflects the population referring to a Memory Clinic. However, it limits the relevance of our results, as the associations described are probably driven by the motor component of the MCR. Further large studies, including cognitively healthy controls, are needed to explore these associations with the cognitive component of MCR. Third, as this was a pilot study and previous works already related PET and MRI features to neuropsychological tests and gait parameters, we did not extend the analysis to such correlations. Lastly, given the design of the study, no inference can be made about the causality of the associations detected.
In conclusion, we found that MCR, especially in its motor component, is associated with lateral ventricular enlargement and microstructural damage of the sCR, but not to amyloid or tau deposits or to global white matter macroscopically detectable damage. Future studies will assess whether MCR is related to a specific spatial pattern of structural disruption, more than the underlying pathogenic pathway, thus helping to better define the predictive impact of MCR in different types of dementia.
ACKNOWLEDGMENTS
We would like to thank all patients for their participation in this study. We thank Marta Martins for her help with the patients’ recruitment.
This work was supported by the Swiss National Science Foundation (SNSF grant #320030_173153, 320030_185028) and the Geneva University Hospitals (PRD grant #8-2019-II). Clinical data for this study were collected at the Centre de la mémoire, Geneva University and University Hospitals, thanks to funds from: Association Suisse pour la Recherche sur l’Alzheimer, Genève; Fondation Segré, Genève; Ivan Pictet, Genève; Fondazione Agusta, Lugano; Fondation Chmielewski, Genève; Velux Stiftung; Swiss National Science Foundation (projects #320030_182772, 320030_169876); Horizon 2020 (projects n. 667375); Human Brain Project; Innovative Medicines Initiatives (IMI contract n. 115736 and 115952).
Authors’ disclosures available online (https://www.j-alz.com/manuscript-disclosures/21-5461r3).
SUPPLEMENTARY MATERIAL
[1] The supplementary material is available in the electronic version of this article: https://dx.doi.org/10.3233/JAD-215461.
REFERENCES
[1] | Verghese J , Wang C , Lipton RB , Holtzer R ((2013) ) Motoric cognitive risk syndrome and the risk of dementia. J Gerontol A Biol Sci Med Sci 68: , 412–418. |
[2] | Meiner Z , Ayers E , Verghese J ((2020) ) Motoric cognitive risk syndrome: A risk factor for cognitive impairment and dementia in different populations. Ann Geriatr Med Res 24: , 3–14. |
[3] | Doi T , Verghese J , Shimada H , Makizako H , Tsutsumimoto K , Hotta R , Nakakubo S , Suzuki T ,((2015) ) Motoric cognitive risk syndrome: Prevalence and risk factors in Japanese seniors. J Am Med Dir Assoc 16 : .1103e21–1103.e25. |
[4] | Beauchet O , Sekhon H , Barden J , Liu-Ambrose T , Chester VL , Szturm T , Grenier S , Léonard G , Bherer L , Allali G , Consortium CG ((2018) ) Association of motoric cognitive risk syndrome with cardiovascular disease and risk factors: Results from an original study and meta-analysis. J Alzheimers Dis 64: , 875–887. |
[5] | Bortone I , Griseta C , Battista P , Castellana F , Lampignano L , Zupo R , Sborgia G , Lozupone M , Moretti B , Giannelli G , Sardone R , Panza F ((2021) ) Physical and cognitive profiles in motoric cognitive risk syndrome in an older population from Southern Italy. Eur J Neurol 28: , 2565–2573. |
[6] | Blumen HM , Allali G , Beauchet O , Lipton RB , Verghese J ((2019) ) A gray matter volume covariance network associated with the motoric cognitive risk syndrome: A multicohort MRI study. J Gerontol A Biol Sci Med Sci 74: , 884–899. |
[7] | Blumen HM , Schwartz E , Allali G , Beauchet O , Callisaya M , Doi T , Shimada H , Srikanth V , Verghese J ((2021) ) Cortical thickness, volume, and surface area in the motoric cognitive risk syndrome.J Alzheimers Dis 81: , 651–665. |
[8] | Sekhon H , Allali G , Launay CP , Barden J , Szturm T , Liu-Ambrose T , Chester VL , Wong CH , Beauchet O ((2019) ) Motoric cognitive risk syndrome, incident cognitive impairment and morphological brain abnormalities: Systematic review and meta-analysis. Maturitas 123: , 45–54. |
[9] | Verghese J , Annweiler C , Ayers E , Barzilai N , Beauchet O , Bennett DA , Bridenbaugh SA , Buchman AS , Callisaya ML , Camicioli R , Capistrant B , Chatterji S , De Cock AM , Ferrucci L , Giladi N , Guralnik JM , Hausdorff JM , Holtzer R , Kim KW , Kowal P , Kressig RW , Lim JY , Lord S , Meguro K , Montero-Odasso M , Muir-Hunter SW , Noone ML , Rochester L , Srikanth V , Wang C ((2014) ) Motoric cognitive risk syndrome Multicountry prevalence and dementia risk. Neurology 83: , 718–726. |
[10] | Beauchet O , Sekhon H , Launay CP , Rolland Y , Schott AM , Allali G ((2020) ) Motoric cognitive risk syndrome and incident dementia: Results from a population-based prospective and observational cohort study. Eur J Neurol 27: , 468–474. |
[11] | Meiner Z , Ayers E , Bennett DA , Wang C , Verghese J ((2021) ) Risk factors for the progression of motoric cognitive risk syndrome to dementia: Retrospective cohort analysis of two populations. Eur J Neurol 28: , 1859–1867. |
[12] | Landau SM , Marks SM , Mormino EC , Rabinovici GD , Oh H , O’Neil JP , Wilson RS , Jagust WJ ((2012) ) Association of lifetime cognitive engagement and low β-amyloid deposition. Arch Neurol 69: , 623–629. |
[13] | Mishra S , Gordon BA , Su Y , Christensen J , Friedrichsen K , Jackson K , Hornbeck R , Balota DA , Cairns NJ , Morris JC , Ances BM , Benzinger TLS ((2017) ) AV-1451 PET imaging of tau pathology in preclinical Alzheimer disease: Defining a summary measure. Neuroimage 161: , 171–178. |
[14] | Klunk WE , Koeppe RA , Price JC , Benzinger TL , Devous MDS , Jagust WJ , Johnson KA , Mathis CA , Minhas D , Pontecorvo MJ , Rowe CC , Skovronsky DM , Mintun MA ((2015) ) The Centiloid Project: Standardizing quantitative amyloid plaque estimation by PET. Alzheimers Dement 11: , 1–15.e1-4. |
[15] | van der Holst HM , Tuladhar AM , Zerbi V , van Uden IWM , de Laat KF , van Leijsen EMC , Ghafoorian M , Platel B , Bergkamp MI , van Norden AGW , Norris DG , van Dijk EJ , Kiliaan AJ , de Leeuw FE ((2018) ) White matter changes and gait decline in cerebral small vessel disease. Neuroimage Clin 17: , 731–738. |
[16] | Maltais M , de Souto Barreto P , Perus L , Mangin JF , Grigis A , Chupin M , Bouyahia A , Gabelle A , Delrieux J , Rolland Y , Vellas B , Multidomain Alzheimer Preventive Trial/Data Sharing Alzheimer (MAPT/DSA) Study Group ((2020) ) Re: Prospective associations between diffusion tensor imaging parameters and frailty in older adults. J Am Geriatr Soc 68: , 1050–1055. |
[17] | Jack CR , Wiste HJ , Weigand SD , Therneau TM , Lowe VJ , Knopman DS , Gunter JL , Senjem ML , Jones DT , Kantarci K , Machulda MM , Mielke MM , Roberts RO , Vemuri P , Reyes DA , Petersen RC ((2017) ) Defining imaging biomarker cut points for brain aging and Alzheimer’s disease. Alzheimers Dement 13: , 205–216. |
[18] | Crook JE , Gunter JL , Ball CT , Jones DT , Graff-Radford J , Knopman DS , Boeve BF , Petersen RC , Jack CR , Graff-Radford NR ((2020) ) Linear vs volume measures of ventricle size. Neurology 94: , e549 LP–e556. |
[19] | Wennberg AMV , Savica R , Mielke MM ((2017) ) Association between various brain pathologies and gait disturbance. Dement Geriatr Cogn Disord 43: , 128–143. |
[20] | Annweiler C , Beauchet O , Bartha R , Montero-Odasso M ((2013) ) Slow gait in MCI is associated with ventricular enlargement: Results from the Gait and Brain Study. J Neural Transm 120: , 1083–1092. |
[21] | Gomez GT , Gottesman RF , Gabriel KP , Palta P , Gross AL , Soldan A , Albert MS , Sullivan KJ , Jack CR , Knopman DS , Windham BG , Walker KA ((2022) ) The association of motoric cognitive risk with incident dementia and neuroimaging characteristics: The Atherosclerosis Risk in Communities Study. Alzheimers Dement 18: , 434–444. |
[22] | Ossenkoppele R , Smith R , Mattsson-Carlgren N , Groot C , Leuzy A , Strandberg O , Palmqvist S , Olsson T , Jögi J , Stormrud E , Cho H , Ryu YH , Choi JY , Boxer AL , Gorno-Tempini ML , Miller BL , Soleimani-Meigooni D , Iaccarino L , La Joie R , Baker S , Borroni E , Klein G , Pontecorvo MJ , Devous MD , Jagust WJ , Lyoo CH , Rabinovici GD , Hansson O ((2021) ) Accuracy of tau positron emission tomography as a prognostic marker in preclinical and prodromal Alzheimer disease: A head-to-head comparison against amyloid positron emission tomography and magnetic resonance imaging. JAMA Neurol 78: , 961–971. |
[23] | Wang N , Allali G , Kesavadas C , Noone ML , Pradeep VG , Blumen HM , Verghese J ((2016) ) Cerebral small vessel disease and motoric cognitive risk syndrome: Results from the Kerala-Einstein Study. J Alzheimers Dis 50: , 699–707. |
[24] | Fries W , Danek A , Scheidtmann K , Hamburger C ((1993) ) Motor recovery following capsular stroke: Role of descending pathways from multiple motor areas. Brain 116: , 369–382. |
[25] | Rosario BL , Rosso AL , Aizenstein HJ , Harris T , Newman AB , Satterfield S , Studenski SA , Yaffe K , Rosano C ((2016) ) Cerebral white matter and slow gait: Contribution of hyperintensities and normal-appearing parenchyma. J Gerontol A Biol Sci Med Sci 71: , 968–973. |
[26] | Nomi JS , Schettini E , Broce I , Dick AS , Uddin LQ ((2018) ) Structural connections of functionally defined human insular subdivisions. Cereb Cortex 28: , 3445–3456. |
[27] | Cauley KA , Cataltepe O ((2014) ) Axial diffusivity of the corona radiata correlated with ventricular size in adult hydrocephalus. Am J Roentgenol 203: , 170–179. |
[28] | Wardlaw JM , Valdés Hernández MC , Muñoz-Maniega S ((2015) ) What are white matter hyperintensities made of? Relevance to vascular cognitive impairment. J Am Heart Assoc 4: , 001140. |
[29] | Kamiya K , Hori M , Irie R , Miyajima M , Nakajima M , Kamagata K , Tsuruta K , Saito A , Nakazawa M , Suzuki Y , Mori H , Kunimatsu A , Arai H , Aoki S , Abe O ((2017) ) Diffusion imaging of reversible and irreversible microstructural changes within the corticospinal tract in idiopathic normal pressure hydrocephalus. Neuroimage Clin 14: , 663–671. |